High-humidity environments and the risk of COVID-19 transmission
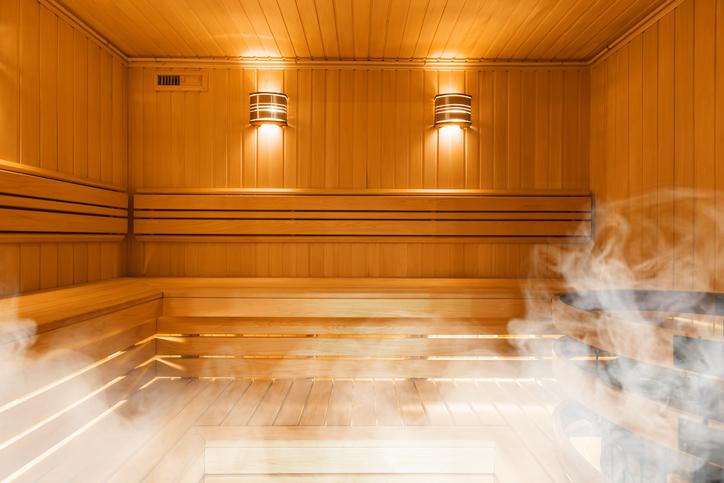
Primary Inquiry: Do showers, steam rooms, hot tubs, or other warm, high-humidity settings in public facilities increase the risk of COVID-19 transmission?
Summary Statement: This rapid review did not identify an elevated transmission risk for showers, steam rooms, or hot tubs as a result of high temperature (>30°C) and/or high humidity (>80% relative humidity). Based on the available data, high relative humidity and high temperature appear to increase airborne mass deposition and decrease the viability of virus in both airborne particles and on surfaces. However, there is uncertainty as to whether SARS-CoV-2 aerosolized in human secretions may remain viable longer than those generated artificial media. In addition, any decrease in viability does not alleviate the need to maintain physical distancing, as well as adequate cleaning, disinfection, and ventilation (where appropriate).
Disclaimer: The information provided here is for the purpose of addressing a specific inquiry related to an environmental health issue. This is not a comprehensive evidence review and has not been subjected to peer review. The information offered here does not supersede federal, provincial or local guidance or regulations, and/or the advice of a medical professional (where applicable).
Background
The COVID-19 pandemic has required public health practitioners to closely scrutinize all publicly accessible indoor environments for characteristics that may help or hinder virus transmission. The primary risk factors for transmission in indoor spaces are crowding, prolonged interactions, common touch surfaces, poor ventilation and user behaviors like intense activity, heavy breathing or loud vocalization.1 Public facilities like steam rooms, locker room showers, and hot tubs share all of these features and raise additional concerns regarding the unknown effects of warmth and very high humidity on the potential for transmission. Although a number of outbreaks have been identified in gyms and fitness facilities,2–5 there is insufficient information to assess whether sharing a high-humidity environment in that facility(rather than just close contact) played a significant role in these events.
Although relative humidity (RH) has long been known to affect the risk of viral transmission, the current recommendations for indoor spaces (maintaining 40-60% RH) are determined by user comfort and the need to control mould.6 The challenge in evaluating facilities like showers, steam rooms, and hot tubs is the relative lack of information on high-humidity environments, as well as the general limitation on literature specific to SARS-CoV-2.
For the purposes of this inquiry, we assumed a scenario in which two or more patrons are sharing the same indoor space, such as a communal shower in a locker room, with no partitions or barriers between them. The question then is whether these patrons would be more or less at risk of transmission simply by turning on the shower. In addition, not all high-humidity settings are equal, but may range in values as shown in Table 1.
Table 1. Typical temperature and relative humidity values for gym facilities.
Facility |
Temperature (°C) |
Relative Humidity (%) |
Shower room |
35 |
100 |
Steam room |
43 |
100 |
Hot tub |
30a |
50-60b |
Dry sauna |
>70 |
5-10 |
aAir temperature of a typical indoor pool deck.
bCode requirement for relative humidity for an indoor pool.
NCCEH addressed this question using the following multi-pronged approach:
- Expert consultation with indoor air quality and environmental health specialists regarding the likelihood of effects;
- A rapid review of academic and NCCEH resources to identify current research pertinent to high-humidity environments;
- A grey literature search for existing guidelines or resources on these settings.
Expert Consultation
Through an informal consultation with indoor air quality and environmental health specialists at the NCCEH and the BC Centre for Disease Control, several potential modes of transmission were identified:
- Close confines in showers, steam rooms and hot tubs make physical distancing difficult; as a result, close-range droplet transmission while speaking or coughing would remain the most important means of transmission. Users may also vocalize louder in a noisy environment, increasing droplet generation.7 High humidity could affect the likelihood of transmission through effects on droplet generation in the upper respiratory tract and effects on droplet dynamics (changes in droplet size and subsequent changes in viability, settling, and range of dispersal).8 Humidity could therefore also affect the formation of smaller particles and the likelihood of short-range aerosol transmission.
- Fomite transmission through high-touch surfaces could occur, as SARS-CoV-2 persists longer on non-porous surfaces, such as ceramic tile and steel faucets. In addition, the temperatures in and around showers, whirlpools, jacuzzis, and steam rooms are not high enough to eliminate the virus instantaneously (with the potential exception of dry saunas).
- Finally, although very unlikely, it may be possible to aerosolize virus from the surfaces previously used by infected individuals.
Rapid Review of Academic Literature
A rapid literature review was conducted to ascertain the effects of high humidity on SARS-CoV-2, with consideration for the potential modes of transmission noted above. Articles were identified using key words related to the effects of relative humidity on the virus itself (SARS-CoV-2, COVID-19, 2019-ncov, coronavirus, viability, infectivity, inactivation, transmission) and the association of high-humidity environments (steam room, shower, locker room, whirl pool, jacuzzi, or hot tubs) with outbreaks, infections, or transmission. EBSCOhost, PubMed, Web of Science, and Google Scholar were used to search for articles.
Over 200 documents were reviewed for title and abstract. Articles were included if they dealt with SARS-CoV-2 (or a reasonable surrogate) in an indoor environment. Topic areas included the effect of high humidity (>70%) on droplet generation; virus loading into droplets; droplet dynamics; and virus viability/inactivation. Bathhouses or other facilities that allow close contact without personal protective equipment were excluded. Studies addressing the effect of outdoor environmental/meteorological conditions on the epidemiology of COVID-19 were not included. Non-peer-reviewed pre-prints were included in the results. In total, 19 studies were identified that were relevant to the effect of humidity on virus transmission in indoor environments.
Does high RH affect droplet dynamics or SARS-CoV2 viability in aerosols?
There are a number of ways in which humidity could potentially affect the presence of infectious droplets: through the formation of droplets in the airway, the partitioning of virus into droplets, the viability of the virus over time, the evaporation of droplets to form droplet nuclei, and the settling of droplets.
This rapid literature search was unable to determine whether inhaling highly humid air (>90% RH) alters either the size or number of respiratory particles produced in the airway, while either vocalizing or breathing. However, COVID-19 patients do produce respiratory particles containing viable SARS-CoV-2. Recent studies have isolated viable SARS-CoV-2 from air samples obtained in a hospital setting. Lednicky et al.9 detected viable SARS-CoV-2 more than 2 m away from the patient to whom it was linked via genome sequencing. Santarpia et al.10 also detected SARS-CoV-2 RNA in several particle fractions (> 4 mm, 1-4 mm, and < 1 mm), and viable virus was recovered from three samples of the smallest fraction.
These studies demonstrate that viable SARS-CoV-2 can be captured from air; however, there is insufficient evidence to understand how extreme changes in humidity affect SARS-CoV-2 viability over time. Schuit et al.11 found that increases in RH alone (from 20% to 70% at 20°C) did not significantly affect the degradation of SARS-CoV-2 aerosolized in simulated saliva or culture medium. This work has been used to create a calculator to estimate the half-life of airborne SARS-CoV-2 under varying conditions; however, the model has insufficient data to estimate decay at high temperatures and RH values.
To complicate matters, previous work on other respiratory viruses over a wider range of RH values has shown that viruses in respiratory particles may be differentially affected by high RH and/or by experimental conditions. Noti et al.12 found that aerosolized influenza virus retains greater viability at low RH and greatly decreased viability at RH values between 40 and 73%.12 Similarly, both van Dormalen et al.13 and Kim et al.14 found that the MERS and transmissible gastroenteritis virus (TGEV), respectively, showed a drastic decrease in viability when RH was increased from 30 through to 90%. These studies would appear to suggest the high RH values are detrimental to coronavirus survival.
However, Lin and Marr15 found that bacteriophages aerosolized in cell culture medium showed decreased viability as RH increased to moderate levels (50-70%), but showed little or no change in viability at high RH values. This was attributed to the much slower rate of evaporation at RH values near saturation, which prevents the concentration of solutes harmful to the virus. This differential effect in response to changes in RH may be at least partly due to the matrix in which the aerosol is created. Kormuth et al.16 found that bacteriophage aerosolized in cell culture medium showed a similar response to increasing RH, as also observed by Lin and Marr.15 However, the same virus aerosolized in a medium containing extracellular matrix from airway cells showed no change or loss in viability across the range of RH values.16 Similarly, SARS-CoV-2 aerosolized in tissue culture medium was more stable at moderate RH (40-60%) than at high RH (68-88%), but much more stable at high RH than at moderate RH when aerosolized in artificial saliva.17 These studies suggest that respiratory secretions have protective effects on aerosolized viruses, and that naturally produced virus aerosols may retain greater infectivity than anticipated.
Humidity also affects the rate of evaporation, the size of respiratory particles, and the time it ultimately requires them to settle (or the distance they may travel as droplet nuclei or aerosols).18,19 Busco et al.20 modeled evaporation and mass deposition from sneeze droplets and found high RH (95%) resulted in greater particle mass deposition than the moderate (65%) or low (35%) RH conditions over the same temperature (35°C) and window of time (50 s). Greater removal of mass at high RH values implies that less material remains to evaporate and form aerosols, lessening the risk of both droplet and aerosol transmission.
From the literature, it appears that higher RH values in showers, steam rooms, and hot tubs may decrease the risk of virus transmission by three means: decreasing the viability of the virus over time, increasing the airborne mass that settles on surface, and decreasing the fraction of droplets that are converted into aerosols. However, there are three important caveats. First, the effect of high humidity on the viability of viral aerosols created by living humans (in respiratory secretions) needs further investigation. Second, virus that settles may still be viable, which may increase the risk of fomite transmission. Third, although high RH values will increase the rate of settling, this will not be sufficient to protect people who are near enough for close-range droplet transmission. Air cleaning technologies like filtration and UV irradiation are impossible to deploy or show reduced effectiveness in high-humidity environments.6 Therefore, the only means to protect users of these facilities is to reduce crowding/interaction, as well as to increase ventilation where applicable (i.e., showers, but not steam rooms).
Does high humidity affect the viability of SARS-CoV-2 deposited on surfaces?
Several studies have examined the persistence of SARS-CoV-2 on a variety of porous and non-porous surfaces at moderate temperature and RH values.21–23 However, only one study has examined the interplay of high humidity and high temperature on SARS-CoV-2. In this study, the virus was suspended in simulated saliva, dotted onto stainless steel, plastic, or nitrile gloves, and incubated at various temperatures (24, 28, and 35°C) and RH values (20, 40, 60, and 80%).24 In droplets held at 24°C, the virus half-life dropped from 15 hours (h) at 20% RH to 8.3 h at 80% RH. In droplets held at 35°C, the half-life dropped from 7.33 h at 20% RH to just 2.26 h at 60%.[1]
These results are consistent with other coronaviruses examined at moderate to high RH values. When held at 40°C, both TGEV and mouse hepatitis virus (MHV), which were suspended in cell culture medium and dotted onto stainless steel, were detectable for at least 120 h at an RH of 20%, compared to only 6 h at an RH of 80%.25 Similarly, SARS-CoV-1 degraded most rapidly when incubated at both a high temperature (38°C) and the highest RH (95%).26 Increasing the RH from 30 to 80% also increased the decay rate for MERS coronavirus incubated on plastic and steel at 30C for 72 h.13 These results seem to suggest that both increasing temperature and increasing RH serve to reduce the viability or infectivity of coronaviruses on nonporous surfaces.
Guillier et al.27 used data from 10 different coronaviruses to create a model that estimates the time required to achieve specific log reductions in viral infectivity at a given temperature and humidity. For example, for virus trapped in droplets deposited on the steel faucet of a communal shower (Table 1: 35°C and 100% RH), their model indicates that it would require approximately 73 h to see a 5-log reduction in virus infectivity. Similarly, for a steam room (Table 1: 43°C and 100% RH), it would take approximately 16 h to reach a 5-log reduction. The model demonstrates that temperature increases have a greater negative impact on virus infectivity than changes in RH, but that both promote virus inactivation.
This model would seem to suggest that patrons using showers, steam rooms, and hot tubs are at a lesser risk of transmission via fomites than when using other facilities in the building held at lower temperatures and lower RH values. However, this model is based on data collected at RH values up to 68% RH. Using this model to estimate log reductions at high values (100% RH) introduces uncertainty into the results. Furthermore, it should be noted however that these studies were carried out in tissue culture media rather than in artificial fluids.
However, work on influenza A virus (IAV)28 and other indicator viruses15 has shown that viruses deposited on surfaces at very high RH values (>80%) do not show a marked decrease in viability compared to viruses incubated at RH values <30%. In fact, virus infectivity shows a U-shaped curve, with the greatest inactivation at moderate RH values in the range of 50 to 80%. The authors postulated that the rate of evaporation, and the time for which the virus is exposed to increasing solute concentrations, are key to virus inactivation.15 At low RH, rapid evaporation results in crystallization of the harmful solutes, protecting the virus from damage. As RH increases, and the rate of evaporation decreases, longer exposures to harmful solutes begin to inactivate the virus. However, at very high RH values, evaporation is slow enough that solute concentrations are not harmful to the virus, allowing them to remain viable.28 Thus, in a very humid environment, some viruses may remain infectious for longer periods than at lower RH values. Further work is required to understand whether SARS-CoV-2 follows the expected trend, decreasing viability with increase temperature and RH, or whether it may show increased viability at very high RH values, as with influenza A.
At best, however, it appears that coronaviruses deposited as droplets on surfaces remain viable for at least several hours. Although this rate of degradation is much more rapid than that expected at lower temperatures and relative humidity, it is not rapid enough to protect other users occupying the space throughout the day.
How might high or low RH values affect individual susceptibility to transmission?
It should also be noted that, in addition to effects on droplet dynamics, low humidity increases the vulnerability of the nasal mucosa to infection by decreasing mucociliary clearance and the activity of innate immune cells.29,30 Thus, we would not expect to see increased vulnerability to infection in a highly humid environment. Although dry saunas are likely to adversely affect the nasal mucosa, it has been shown that high temperatures (>41°C) result in disruption or disordering of lipids in the viral envelope of influenza.31 Indeed, coronaviruses (including SARS-CoV-2) show a rapid decline in viability as temperatures increase from 20°C to 68°C.27 Using the model provided by Guillier et al.,27 we can estimate that it would take only 0.01 h to reach a 5-log reduction in coronavirus viability for droplets deposited in a dry sauna (Table 1: 70°C and 10% RH). This effectively negates the possibility of fomite spread in dry saunas and likely also the survival of aerosols, although the possibility of direct contact or close-range droplets (transmitting over a few seconds) remains.
Other means of aerosolization: spray attachments and contaminated drains
The persistence of infectious material deposited on surfaces at high RH values indicates the need for more frequent cleaning of high-humidity settings like showers and steam rooms. However, it may be prudent to avoid cleaning and disinfection practices that could re-aerosolize virus from deposited droplets (e.g., use of high-pressure hoses or spray attachments). When cleaning, one option may be to apply a Health Canada-approved disinfectant first, allow sufficient time for virus inactivation, and then remove with running water. Appropriate personal protective equipment (mask and gloves) should be used according to the manufacturer’s label. It is important to note that certain disinfectant chemicals may cause adverse health effects for people with asthma, allergies, or other respiratory conditions. Precautions should be taken when selecting the appropriate type of disinfectant and application method to avoid harm to the user and others.32
Previously, there has been substantial media attention paid to aerosolization of coronaviruses from plumbing into inhabited spaces. This occurred at the Amoy Gardens housing complex during the Hong Kong SARS outbreak in 2003,33 and more recently may have occurred again with SARS-CoV-2 in apartment towers in Hong Kong34 and Guangzhou.35 SARS-CoV-2 has also be detected in the drain water from both sinks and showers in households where ill people were in isolation.36 However, it should be noted that previous incidents in which virus was aerosolized from plumbing involved a critical fault or failure; e.g., a dry drain trap, faulty venting, etc. In the case of a communal shower in a public facility, larger diameter plumbing and high flows of water make it highly unlikely that the virus could accumulate within or be drawn back into the shower room. No evidence has been found to date indicating that such an outbreak would be likely.
Guidance for managing high-humidity environments
A search for North American guidance documents mentioning showers, steam rooms, or hot tubs (including spa pools, jacuzzis, and whirlpools) revealed numerous jurisdictions that have permitted showers to re-open, but specifically prohibit dry saunas, steam rooms, and hot tubs. Most recently, the Government of Alberta has allowed indoor showers, hot tubs, whirlpools, steam rooms, and dry saunas to re-open, but requires more frequent cleaning and that facilities provide a spray bottle of disinfectant for each patron to use after showering.37,38 Their guidance also notes that masks should not be worn in high humidity environments, as they are ineffective when damp and may pose additional safety risks.39 No other specific guidance is available on managing high-humidity environments at this time.
Summary
This rapid review of the literature did not identify an elevated transmission risk for showers, steam rooms, or hot tubs as a result of high humidity (>80%). In fact, the available data appear to suggest that high RH values will decrease airborne material and decrease the viability of virus in airborne particles and on surfaces, although this does not alleviate the need to maintain physical distancing, as well as adequate cleaning and disinfection practices.
Acknowledgements
This document benefitted from the contributions of Lydia Ma, Tina Chen, Juliette O’Keeffe, Michelle Wiens, and Tom Kosatsky (NCCEH), as well as Sarah Henderson (BC Centre for Disease Control.
[1] The half-life at 80% RH could not be evaluated due to equipment limitations.
References
- Chen T, O'Keeffe J. COVID-19 in indoor environments — Air and surface disinfection measures [guidance document]. Vancouver, BC: National Collaborating Centre for Environmental Health; 2020 Jul. Available from: https://ncceh.ca/documents/guide/covid-19-indoor-environments-air-and-surface-disinfection-measures.
- Leclerc QJ, Fuller NM, Knight LE, Funk S, Knight GM. What settings have been linked to SARS-CoV-2 transmission clusters? Wellcome Open Research. 2020;5:83-. Available from: https://doi.org/10.12688/wellcomeopenres.15889.1.
- Luo C, Yao L, Zhang L, Yao M, Chen X, Wang Q, et al. Possible transmission of severe acute respiratory syndrome coronavirus 2 (SARS-CoV-2) in a public bath center in Huai'an, Jiangsu Province, China. JAMA Netw Open. 2020 Mar 2;3(3):e204583. Available from: https://doi.org/10.1001/jamanetworkopen.2020.4583.
- Jang S, Han SH, Rhee J-Y. Cluster of coronavirus disease associated with fitness dance classes, South Korea. Emerg Infect Dis. 2020;26(8):1917-20. Available from: https://wwwnc.cdc.gov/eid/article/26/8/20-0633_article.
- Brlek A, Vidovič S, Vuzem S, Turk K, Simonović Z. Possible indirect transmission of COVID-19 at a squash court, Slovenia, March 2020: Case report. Epidemiol Infect. 2020;148. Available from: https://doi.org/10.1017/S0950268820001326.
- Ashrae Epidemic Task Force. ASHRAE position document on infectious aerosols. Atlanta, GA2020. Available from: https://www.ashrae.org/file%20library/about/position%20documents/pd_infectiousaerosols_2020.pdf.
- Stadnytskyi V, Bax CE, Bax A, Anfinrud P. The airborne lifetime of small speech droplets and their potential importance in SARS-CoV-2 transmission. Proc Nat Acad Sci USA. 2020 Jun;117(22):11875-7. Available from: https://www.pnas.org/content/117/22/11875.
- Wei J, Li Y. Airborne spread of infectious agents in the indoor environment. Am J Infect Control. 2016;44(9):S102-S8. Available from: https://doi.org/10.1016/j.ajic.2016.06.003.
- Lednicky JA, Lauzardo M, Fan ZH, Jutla A, Tilly TB, Gangwar M, et al. Viable SARS-CoV-2 in the air of a hospital room with COVID-19 patients. Int J Infect Dis. 2020 Sep 15. Available from: https://doi.org/10.1016/j.ijid.2020.09.025.
- Santarpia JL, Herrera VL, Rivera DN, Ratnesar-Shumate S, Reid SP, Denton PW, et al. The infectious nature of patient-generated SARS-CoV-2 aerosol. MedRxiv. 2020 Jul 21. Available from: https://www.medrxiv.org/content/10.1101/2020.07.13.20041632v2.
- Schuit M, Gardner S, Wood S, Bower K, Williams G, Freeburger D, et al. The influence of simulated sunlight on the inactivation of influenza virus in aerosols. J Infect Dis. 2019;221(3):372-8. Available from: https://doi.org/10.1093/infdis/jiz582.
- Noti JD, Blachere FM, McMillen CM, Lindsley WG, Kashon ML, Slaughter DR, et al. High humidity leads to loss of infectious influenza virus from simulated coughs. PLoS ONE. 2013;8(2):e57485. Available from: https://doi.org/10.1371/journal.pone.0057485.
- van Doremalen N, Bushmaker T, Munster VJ. Stability of Middle East respiratory syndrome coronavirus (MERS-CoV) under different environmental conditions. Euro Surveill. 2013;18(38). Available from: https://doi.org/10.2807/1560-7917.es2013.18.38.20590.
- Kim SW, Ramakrishnan MA, Raynor PC, Goyal SM. Effects of humidity and other factors on the generation and sampling of a coronavirus aerosol. Aerobiologia. 2007;23(4):239-48. Available from: https://dx.doi.org/10.1007%2Fs10453-007-9068-9.
- Lin K, Marr LC. Humidity-dependent decay of viruses, but not bacteria, in aerosols and droplets follows disinfection kinetics. Environ Sci Tech. 2020;54(2):1024-32. Available from: https://pubs.acs.org/doi/full/10.1021/acs.est.9b04959.
- Kormuth KA, Lin K, Prussin Ii AJ, Vejerano EP, Tiwari AJ, Cox SS, et al. Influenza virus infectivity is retained in aerosols and droplets independent of relative humidity. J Infect Dis. 2018;218(5):739-47. Available from: https://doi.org/10.1093/infdis/jiy221.
- Smither SJ, Eastaugh LS, Findlay JS, Lever MS. Experimental aerosol survival of SARS-CoV-2 in artificial saliva and tissue culture media at medium and high humidity. Emerg Microbes Infect. 2020 Dec;9(1):1415-7. Available from: https://doi.org/10.1080/22221751.2020.1777906.
- Marr LC, Tang JW, Van Mullekom J, Lakdawala SS. Mechanistic insights into the effect of humidity on airborne influenza virus survival, transmission and incidence. J R Soc Interface. 2019;16(150). Available from: https://royalsocietypublishing.org/doi/full/10.1098/rsif.2018.0298.
- Chen LD. Effects of ambient temperature and humidity on droplet lifetime - A perspective of exhalation sneeze droplets with COVID-19 virus transmission. Int J Hyg Environ Health. 2020 Aug;229:113568. Available from: https://doi.org/10.1016/j.ijheh.2020.113568.
- Busco G, Yang SR, Seo J, Hassan YA. Sneezing and asymptomatic virus transmission. Phys Fluids. 2020;32(7):73309-. Available from: https://dx.doi.org/10.1063%2F5.0019090.
- van Doremalen N, Bushmaker T, Morris DH, Holbrook MG, Gamble A, Williamson BN, et al. Aerosol and surface stability of SARS-CoV-2 as compared with SARS-CoV-1. N Engl J Med. 2020;382:1564-7. Available from: https://doi.org/10.1056/NEJMc2004973.
- Chin AWH, Chu JTS, Perera MRA, Hui KPY, Yen H-L, Chan MCW, et al. Stability of SARS-CoV-2 in different environmental conditions. Lancet Microbe. 2020;1(1):e10. Available from: https://doi.org/10.1016/S2666-5247(20)30003-3.
- Fears A, Klimstra W, Duprex P, Hartman A, Weaver S, Plante K, et al. Comparative dynamic aerosol efficiencies of three emergent coronaviruses and the unusual persistence of SARS-CoV-2 in aerosol suspensions. medRxiv. 2020. Available from: https://dx.doi.org/10.1101%2F2020.04.13.20063784.
- Biryukov J, Boydston JA, Dunning RA, Yeager JJ, Wood S, Reese AL, et al. Increasing temperature and relative humidity accelerates inactivation of SARS-CoV-2 on surfaces. mSphere. 2020 Jul 1;5(4). Available from: https://doi.org/10.1128/msphere.00441-20.
- Casanova LM, Jeon S, Rutala WA, Weber DJ, Sobsey MD. Effects of air temperature and relative humidity on coronavirus survival on surfaces. Appl Environ Microbiol. 2010;76(9):2712-7. Available from: https://doi.org/10.1128/aem.02291-09.
- Chan KH, Peiris JSMM, Lam SY, Poon LLMM, Yuen KY, Seto WH. The effects of temperature and relative humidity on the viability of the sars coronavirus. Adv Virol. 2011. Available from: https://doi.org/10.1155/2011/734690.
- Guillier L, Martin-Latil S, Chaix E, Thébault A, Pavio N, Le Poder S, et al. Modeling the inactivation of viruses from the coronaviridae family in response to temperature and relative humidity in suspensions or on surfaces. Appl Environ Microbiol. 2020;86(18):e01244-20. Available from: https://aem.asm.org/content/aem/86/18/e01244-20.full.pdf.
- Yang W, Elankumaran S, Marr LC. Relationship between humidity and Influenza A viability in droplets and implications for influenza’s seasonality. PLoS ONE. 2012;7(10):e46789-e. Available from: https://dx.plos.org/10.1371/journal.pone.0046789.
- Salah B, Dinh Xuan AT, Fouilladieu JL, Lockhart A, Regnard J. Nasal mucociliary transport in healthy subjects is slower when breathing dry air. Eur Respir J. 1988;1(9). Available from: https://erj.ersjournals.com/content/1/9/852.article-info.
- Eccles R. An explanation for the seasonality of acute upper respiratory tract viral infections. Acta Otolaryngol. 2002;122(2):183-91. Available from: https://doi.org/10.1080/00016480252814207.
- Polozov IV, Bezrukov L, Gawrisch K, Zimmerberg J. Progressive ordering with decreasing temperature of the phospholipids of influenza virus. Nat Chem Biol. 2008;4(4):248-55. Available from: https://doi.org/10.1038/nchembio.77.
- National Institute for Occupational Safety and Health. Hazard communication for disinfectants used against viruses. Atlanta, GA: US Centers for Disease Control and Prevention; 2020 Sep. Available from: https://www.cdc.gov/niosh/topics/disinfectant/default.html.
- McKinney K, Gong Y, Lewis T. Environmental transmission of SARS at Amoy Gardens. J Environ Health. 2006;68:26-30; quiz 51. Available from: https://pubmed.ncbi.nlm.nih.gov/16696450/.
- Regan H. How can the coronavirus spread through bathroom pipes? Experts are investigating in Hong Kong. CNN. 2020 Feb 12. Available from: https://www.cnn.com/2020/02/12/asia/hong-kong-coronavirus-pipes-intl-hnk/index.html.
- Tang S, Mao Y, Jones RM, Tan Q, Ji JS, Li N, et al. Aerosol transmission of SARS-CoV-2? Evidence, prevention and control. Environ Int. 2020;144:106039-. Available from: https://dx.doi.org/10.1016%2Fj.envint.2020.106039.
- Döhla M, Wilbring G, Schulte B, Kümmerer BM, Diegmann C, Sib E, et al. SARS-CoV-2 in environmental samples of quarantined households. medRxiv. 2020. Available from: https://www.medrxiv.org/content/medrxiv/early/2020/06/02/2020.05.28.20114041.full.pdf.
- Government of Alberta. Guidance for sport, physical activity and recreation - stage 2. Edmonton, AB: Government of Alberta; 2020 Jul. Available from: https://www.lifesaving.org/public/download/files/133437.
- Government of Alberta. COVID-19 guidance for swimming pools and whirlpools. Edmonton, AB: Government of Alberta; 2020 Sep. Available from: https://www.alberta.ca/assets/documents/covid-19-relaunch-guidance-swimming-pools-and-whirlpools.pdf.
39. O'Keeffe J. Masking during the COVID-19 pandemic. Vancouver, BC: National Collaborating Centre for Environmental Health; 2020 Apr 17. Available from: https://ncceh.ca/documents/guide/masking-during-covid-19-pandemic.